The Dynamics of Electric Vehicle Fires
- April 8, 2024
- 11:49 am
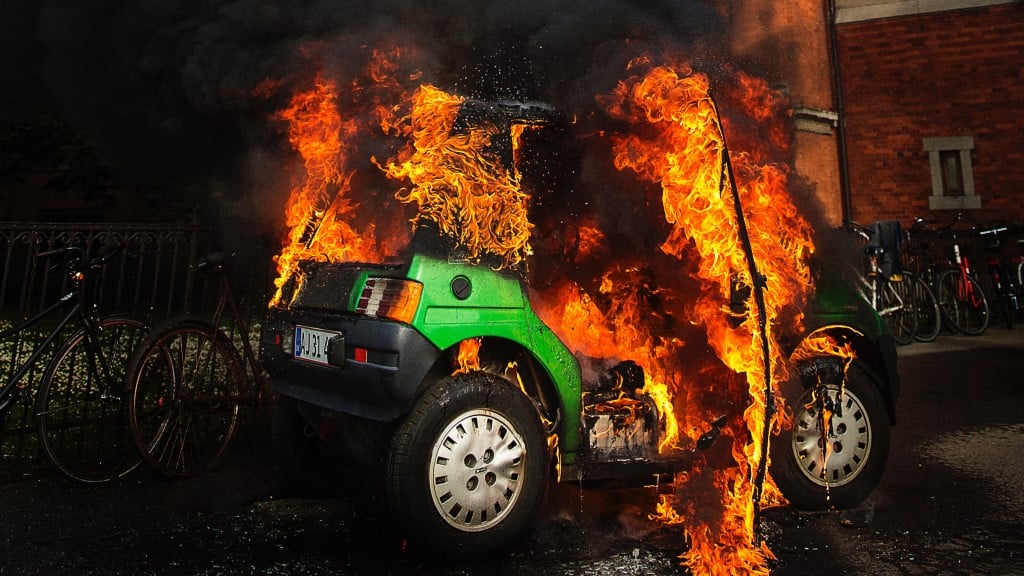
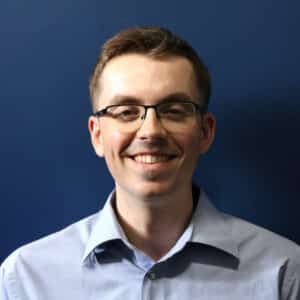
Iain Hoey
Share this content
Jessica Gallo delves into the intricacies of electric vehicle fires, examining their causes, consequences, and the future of EV safety
Electric vehicles (EVs) are a groundbreaking technology that are slowly taking over the world.
A vehicle that can run without fuel combustion or pollution yet may be powerful enough to power a home for days at a time, is the result of decades of investment and research.
However, as these vehicles increase in prevalence, the risk of EV fires also continues to increase with limited available research and education.
Electric Vehicle Fires: An Overview and Analysis aims to help fill the educational gap regarding EVs and EV fires.
EVs date back nearly two centuries to the creation of the first EV motor in 1828.
Despite development efforts in the late 19th century, EVs declined with the rise of internal combustion engines (ICEs), notably the Ford Model T.
Resurgence occurred in the 1970s due to oil dependence concerns, but faced challenges in performance, range, and price.
Tesla’s launch of the Roadster in 2008 marked a turning point, offering long-range luxury EVs.
Advances in battery technology, especially lithium-ion batteries (LIBs), further improved range and reduced costs, leading to a proliferation of EV options from various manufacturers and a significant shift towards affordable, long-range, eco-friendly transportation. 
For large-scale EV adoption, significant infrastructure development needs to take place.
This is occurring through the expansion of EV chargers as well as battery manufacturing plants, signifying the buy-in of consumers, manufacturers, and governmental institutions.
Cost factors
Evaluating the total costs of EVs versus ICE vehicles encompasses aspects such as the costs of charging and fueling, maintenance expenses, and available incentives.
EVs generally command a higher upfront cost but benefit from reduced maintenance and access to potential tax benefits.
The expense of powering EVs as opposed to refueling ICE vehicles fluctuates with the prices of electricity and gasoline, charging practices, and how the vehicle is used.
Charging at home generally offers cost savings, which can vary by location.
Despite the variability in fuel and electricity costs, research indicates that EVs can lead to cost savings over the vehicle’s life due to their lower maintenance needs, and additional improvements to range extending technology (i.e. regenerative braking) increasing vehicle efficiency.
While EV fires are a focus of media attention, they statistically rarely occur.
Although the number of ICE vehicles on the road significantly outnumbers those of EVs, according to data from the National Transportation Safety Board (NTSB), the rate of fires per 100k ICE vehicles on the road is nominally 40-60 times higher than that of EVs.
Thus, the number of ICE fires occurring daily far exceeds that of EV fires, and even with EVs becoming more prevalent, based on the failure rate data, it would be expected that EV fires would occur less frequently.
Thermal runaway
A Thermal Runaway (TR) is a major cause of EV battery fires, triggered by overcharge, short circuit, or other faults, and is an additional cause of fire outside of those experienced by an ICE vehicle.
Fighting EV battery fires can be challenging due to the risk of reignition, stranded energy, and the ability to get suppression agents to the fuel source.
EV safety is a growing field of research and development and is constantly improving.
Numerous safety features are integrated into the design of the EV: battery packs are often installed in reinforced compartments to prevent punctures that could lead to TR and/or fires; active liquid cooling is often used to regulate the battery temperature to avoid thermal effects; and battery management systems are integrated into the EV to monitor and control the health and operating state of the batteries.
There are two primary battery chemistries used in EVs: Lithium Iron Phosphate (LFP) and Lithium Nickel Manganese Cobalt Oxide (NMC).
Cell chemistry will vary by manufacturer, and along with the cell form factor, will impact stability, cost, energy density, charge rate, and life cycle.
Each chemistry and form factor offers several advantages; however, manufacturers are increasingly utilizing LFP cells over NMC in product vehicles.
LFP batteries are noted for their wider temperature range, lower cost, and overall stability, while NMC batteries offer higher energy capacity and nominal voltage.
Safety considerations, particularly regarding TR and venting, highlight advantages in thermal stability and slower venting in LFP batteries compared to NMC batteries.
The future development of battery chemistries will consider factors like safety, range, environmental impact, and resource availability.
Battery fires can propagate rapidly, making cell form factor selection critical for safety. There are three main form factors used in EVS: cylindrical, pouch, and prismatic.
Cylindrical cells have high energy density but lower packing efficiency due to unavoidable space between cells.
Prismatic cells have slightly lower energy density but better packing efficiency.
Cylindrical and prismatic cells can also have integrated vents or other safety features.
Pouch cells are constructed by stacking layers of components into a flexible pouch, offering high energy density and packing efficiency but lacking built-in safety features.
Cylindrical cells have metal casings and limited cell-to-cell contact, that can lower propagation rates compared to pouch cells.
Prismatic cells can resist thermal abuse due to their larger size. Prismatic and pouch cells are predominantly used in EVs.
Based on the requirements of the original equipment manufacturer (OEM), connections may be made in series to increase voltage or in parallel to change electrical characteristics.
Wireless battery connections offer potential advantages in reducing complexity, weight, and allowing for easier reconfiguration of battery packs with standardization.
There continues to be a lack of standardized battery specifications, leading manufacturers to choose batteries and their connections based on specific goals.
Managing battery temperature can happen through cooling methods like passive radiative fire protection, air, and liquid cooling.
Liquid cooling, though the most expensive, is the most effective, offering high heat conductivity and compactness.
Indirect liquid cooling, which occurs by circulating coolant around battery packs, is widely used in EVs due to its efficiency and safety.
Comparison studies show indirect liquid cooling outperforming other methods in temperature control.
However, challenges like corrosion and weight exist because of the extra fluid and unpredictable environmental conditions, thus other methods are still frequently used.
EV’s future
Advancements in EV research focus on safety and efficiency.
Moving the battery inside the vehicle reduces puncture risks, improves temperature control, and aids firefighting access.
Research suggests internal battery placement also improves efficiency, range, agility, and manufacturing costs while reducing environmental impact.
Transitioning to solid-state batteries (SSBs) or cobalt-free/nickel-based alternatives aim to mitigate fire risks associated with liquid-electrolyte LIBs, enhancing safety, but facing challenges in mass production and design complexity.
It must also be noted that though SSBs require higher temperatures to undergo venting and TR compared to standard LIBs, their venting and TR reactions can be significantly more powerful, hazardous, and difficult to extinguish.
Research on higher voltage batteries targets faster charging, lower heat generation, and longer range, meeting consumer demands while reducing overheating risks.
Battery swapping, though promising, encounters obstacles in standardization and infrastructure, particularly in diverse markets like the US.
The paper provides a comprehensive review of EVs, covering their history, functionality, materials, cooling mechanisms, and societal impact.
The conclusion was that the safest and least fire-prone EVs utilize prismatic LFP batteries with indirect liquid cooling.
There is a need for ongoing research in several areas, including battery cooling methods, SSB limits, cobalt-free LIBs, battery location, and charging infrastructure.
Furthermore, it highlights the importance of researching extinguishing methods for EV fires to ensure safety for firefighters and the environment, noting the lack of standardized protocols and the potential environmental and health risks associated with EV fires.